NFI Fellows 2021-2022
BW Fellows 2019-2020
BW Fellows 2018-2019
BW Fellows 2017-2018
Bw Fellows 2016-2017
BW Fellows 2015-2016
BW Fellows 2014-2015
|
2019-2020 Blue Waters Graduate Fellows
The following is a summary of the projects for the 2019-2020 fellows.
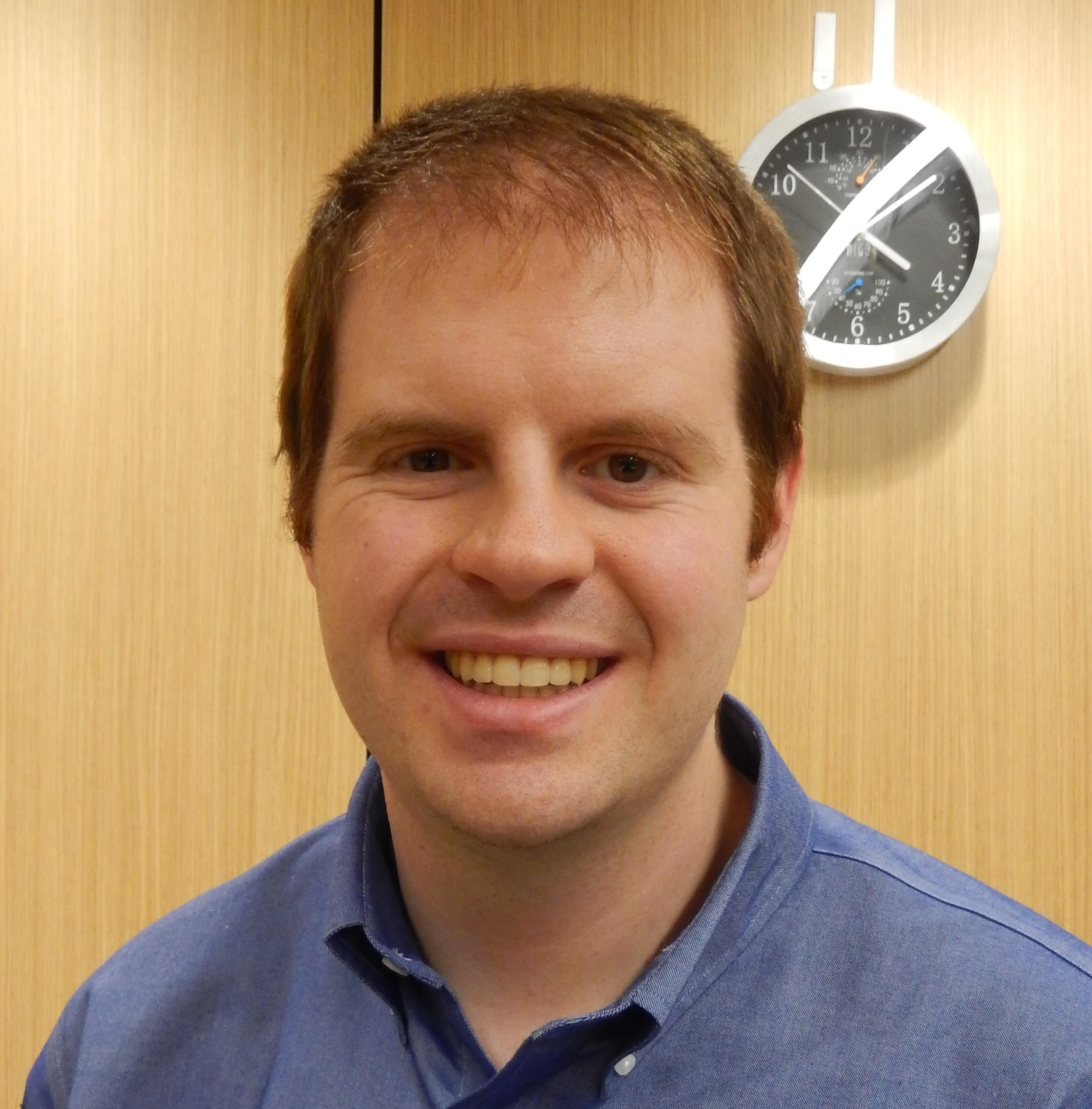 |
Forrest Glines
Michigan State University
While magnetic fields are present in all galaxies, the full nature of their coupling is still debated. The coevolution of galaxies and their magnetic fields can be studied through Magnetohydrodynamic (MHD) models. Higher order and higher resolution MHD methods are needed to provide more physically realistic simulations, but these require efficient usage of large scale computational resources. To explore next generation MHD simulations of galaxies, I will use K-Athena, a CPU and GPU performant version of the MHD code Athena++, to simulate magnetic turbulence in the Taylor-Green vortex. I will use work with K-Athena to inform high resolution galaxy simulations using Enzo-E, a cosmology code built for exascale, with higher order MHD methods.
|
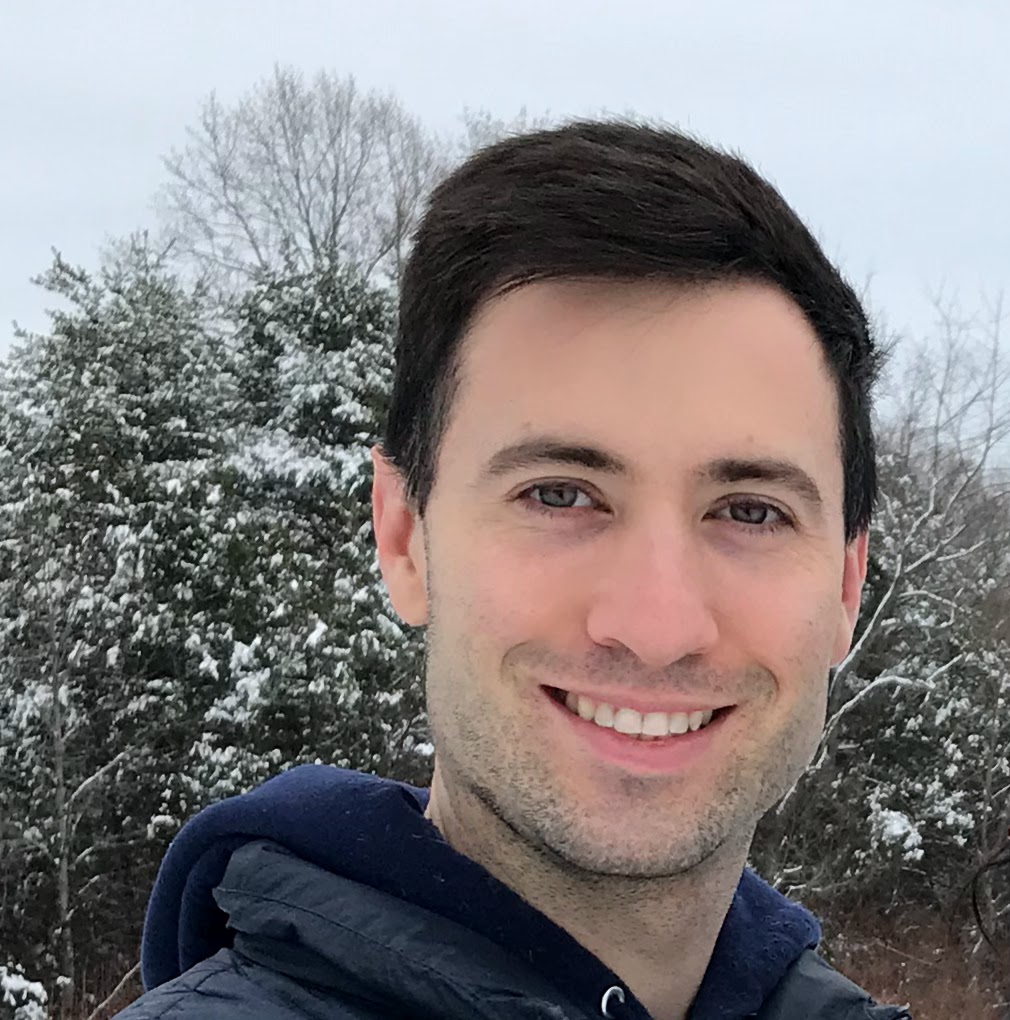 |
Josh Lansford
University of Delaware
The electronic density distribution completely specifies a chemical system's state and can be calculated using density functional theory (DFT). We are developing an atom-centered machine learning (ML) algorithm, trained on electron density, which can generate catalytic reaction mechanisms and kinetic models at reduced computational cost. The ML-based model will accelerate the three most computationally intensive components of the reaction energy profile: transition states, global minima, and entropy. This methodology will be the first to combine structural and density data to enhance ML convergence. Due to the atom-centered nature, the representation of the molecular systems will be invariant to rotations, translations, and reordering of atoms. Because we will use distances, partial charges, and atomic dipoles, the model will be generalizable to any size system.
|
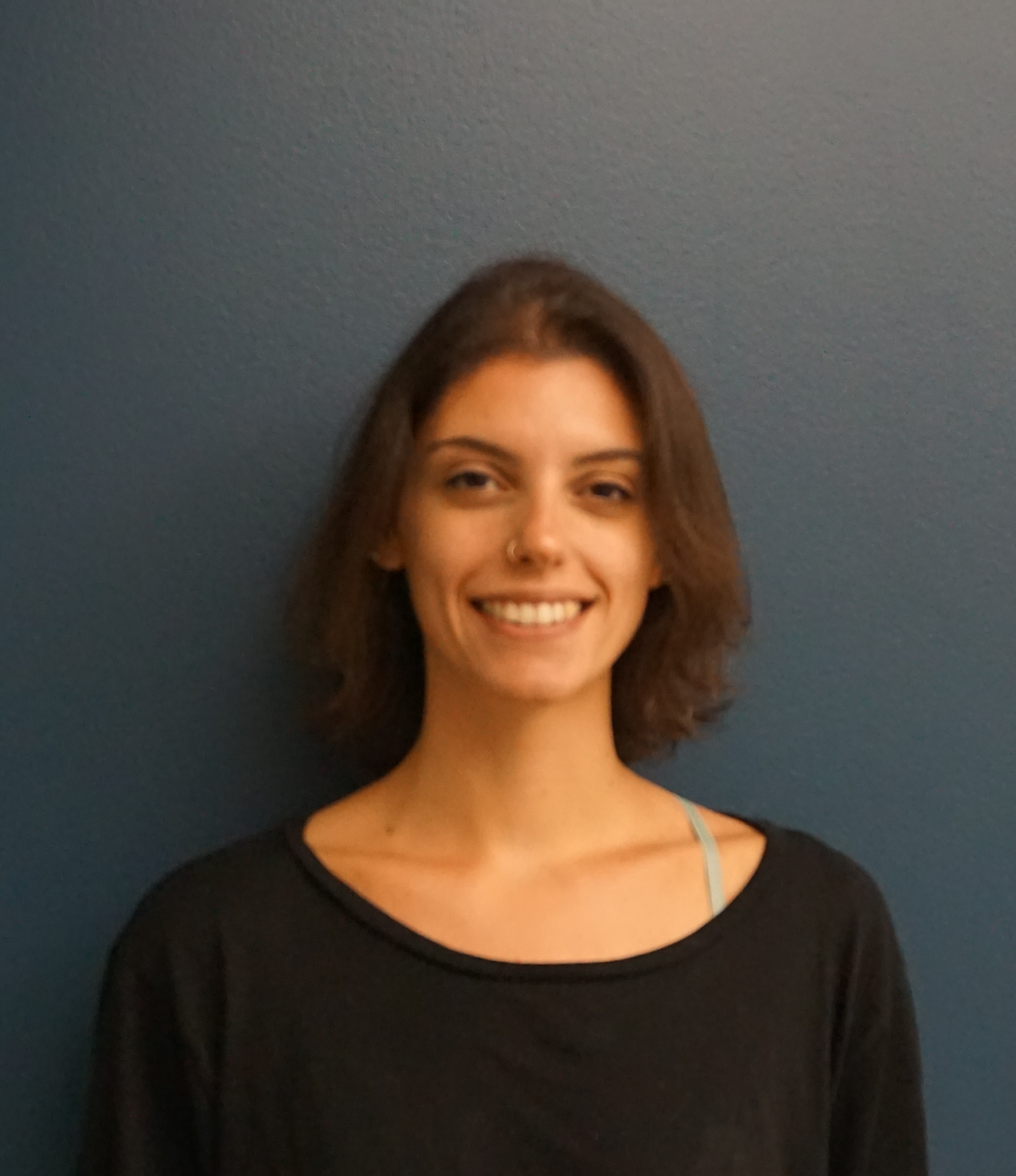 |
Nicole Rosato
Rochester Institute of Technology
In order to solve the General Relativistic field equations on a computer, one must specify the coordinates via the lapse and shift equations. This project studies the effects of new initial values for the lapse and shift. The expressions are constructed using analytic formulas, and mimic their expected settled behavior in the moving puncture gauge. These initial values allow for substantial improvements in the initial shape of both the lapse and the shift. Current simulations set initial shift and initial lapse to be isotropic asymptotically, while our new initial construction of the lapse and shift sets these initial values to be closer to the final, settled shape of the lapse and shift for boosted, spinning black holes. To evaluate the effects of the initial lapse and shift on evolutions, we monitor both the Hamiltonian and Momentum constraint violations. This method shows explicit improvements in preliminary simulations of an unequal-mass, 1:3, nonspinning system and also in an equal-mass system with spins a=0.8. The project proposes to study the more challenging cases of 1:7 mass ratio, and beyond, binary black hole systems with no spin, and highly spinning (a=0.95) systems, which are of utmost interest for observations by the Laser-Interferometer Gravitational-wave Observatory (LIGO). The project will also attempt to improve upon the evolution equations governing the lapse and shift. It is expected that this new method will reduce the amount of time required for the settling of the lapse and shift, and thus will make simulations of both high mass-ratio and near-extremally spinning systems more efficient and accurate.
|
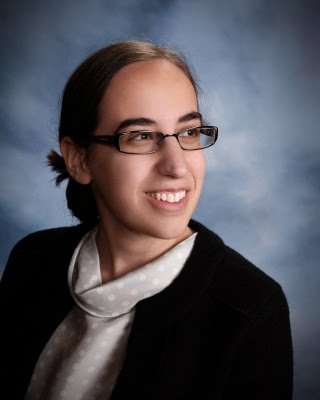 |
Micheline Soley
Harvard University
Ultracold chemistry, in which reactions that occur below one milliKelvin, provide an opportunity for applications from quantum computers to investigations of fundamental constants of nature. The ultracold temperatures of the reactions offer the chance to control reactions at the quantum level. Among ultracold chemical reactions, the ultracold KRb dimer reaction (2KRb → K2 + Rb2) is particularly interesting because of the extreme difference between the ultracold temperature of the incoming reactants and the hot temperature of the colliding atoms. The disparate temperatures give rise to the question of whether classical or quantum effects prevail. Time-dependent quantum dynamical simulations are computationally intensive, so much so that the reaction is difficult to simulate even on supercomputers. Knowledge of whether the reaction can be simulated classically can help bring simulation of ultracold reactions within reach. With the aid of supercomputers, I am exploring the application of complex absorbing potentials and R-matrix theory for the prediction and analysis of the ultracold KRb dimer reaction.
|
|